A Primer on the Sustainable Golden Ticket
Bio-based fibers are the fiber industry’s response to the world’s calls for sustainability. However, the term “bio-based fiber” is often used loosely. In a broad definition, bio-based fibers include any fibers derived of biological origins, which can be divided into three major types: bio-based natural fibers (e.g., wool, cotton, hemp, flax, jute); regenerated fibers (e.g., cellulose, protein, chitosan); and bio-based synthetic fibers (e.g., polylactic acid). But some definitions may exclude one of these three major types – for example, bio-based synthetic fibers are often excluded because they are chemically modified.
Moreover, each major type could have different definitions. For example, some organizations define regenerated fibers as regenerated cellulose fibers (e.g., viscose, modal, lyocell), while some other definitions may also include cellulose-like regenerated fibers (e.g., soybean protein fibers, chitosan fibers) and even modified cellulose fibers (e.g., acetate fiber).
On the other hand, the terms bio-based and biodegradable are often confused or used interchangeably. These two classes, however, are not necessarily mutually inclusive – bio-based fibers are not necessarily biodegradable and vice versa. Briefly speaking, all natural fibers and regenerated fibers are biodegradable, but bio-based synthetic fibers could be biodegradable (e.g., polylactic acid) or non-biodegradable (e.g., bio-based polytrimethylene terephthalate).
This article will provide an overview on the advances of markets and technologies for some of the most prominent bio-based fibers.
Bio-based Natural Fibers
Natural fibers include three product types based on their origins: mineral-, animal-, and plant-based. Animal fibers and plant fibers are bio-based, but mineral fibers (e.g., asbestos) are not. Animal-based natural fibers include animal hair (e.g., wool, cashmere, camel) and cocoon (silk), while plant-based fibers include seed (e.g., cotton), bast (e.g., hemp, jute, flax, ramie), and leaf (e.g., pineapple).
The use of natural fibers began before recorded history. Today the bio-based natural textile fiber market is dominated by cotton with nearly 90% market share, followed by wool, bast and leaf fibers,
and silk.
Cotton alone accounts for nearly one fourth of the global textile fiber production. The International Cotton Advisory Committee (ICAC) estimated the global cotton output was 24.55 million metric tons in 2022-2023, down from 25.18 million metric tons in 2021-2022. However, the future of cotton cultivation is inhibited by problems such as extensive land use, vast amounts of water consumption, and significant use of pesticides and fertilizers. The fiber industry’s answers to these problems include growing organic cotton or bast and leaf fibers that uses less land, water, pesticides, and fertilizers.
Growing organic cotton has made some progress in recent years. According to Textile Exchange, 342,265 metric tons of organic cotton fibers were produced in 2020/21, up 37% from the 2019/20 level, accounting for 1.4% of all cotton grown. However, the overall cotton production is still slow, projected to grow only 1.5% per year to reach 28 million metric tons by 2030, according to the Organization for Economic Cooperation and Development (OECD).
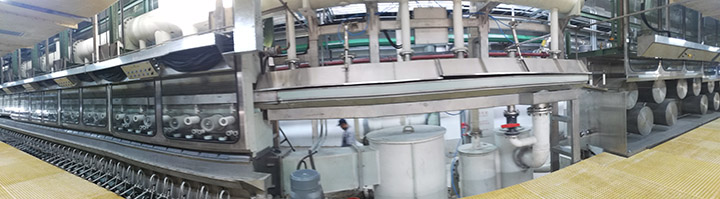
On the other hand, some of the bast and leaf fibers have unique properties and advantages such as being stronger and more resilient, which allow them to be used in a wide range of end applications and create a substantial market size. However, bast and leaf fibers also have disadvantages such as being more difficult to process or spin, more expensive, or less comfortable compared to cotton, which impair their competitiveness against other popular fibers. In addition, the production of bast and leaf fibers also rely heavily on weather, prices, farmers’ economic incentives, and other factors. All these factors reduce the potential of bast and leaf fibers’ contribution to natural fibers’ growth. According to the Food and Agriculture Organization (FAO), total flax fiber and tow production reached an all-time high of approximately 1.5 million metric tons (including both textile and non-textile applications) in 2005 and an all-time low of 0.49 million metric tons in 2011. The average annual growth was only 0.4% from 1961 to 2021.
Regenerated Fibers
Cellulose fibers have a history of more than 160 years. Rayon, developed in 1846 to replace silk, is the world’s first cellulose fiber, and also the first manmade fiber. Cellulose fibers are made from natural cellulose sources, such as cotton pulp and wood pulp, by dissolving them in chemicals and reforming them into fibers. Cellulose, which is part of all major plants, is bio-based and biodegradable, and its degradation does not cause any microplastic particles. Therefore, producers of cellulose fibers are positioning themselves for sustainability claims, which boosted a growth of more than 5% per year in recent years.
However, there are also some hurdles that impede the further growth of cellulose fibers. For example, the raw materials of cellulose fibers rely heavily on wood and cotton, but cotton production stagnates and the cellulose yield from wood is low. On the other hand, there are lack of cradle-to-cradle life cycle analyses that prove the benefits of cellulose – in fact, the standard process for cellulose production may involve washing and bleaching with chlorine, or other chemicals which exacerbate pollutants.
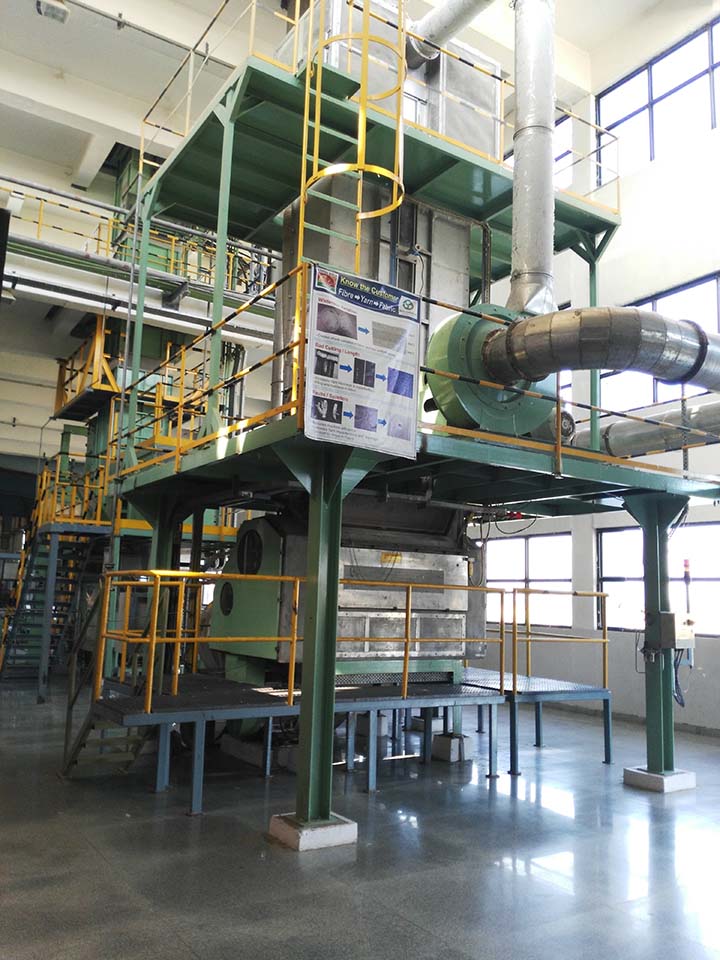
Currently, researchers are finding new solutions to tackle these problems. Manufacturers have done life cycle analyses based on innovated technologies that produce sustainable benefits – for example, energy-efficient processes for cleanly separating cellulose from lignin and hemicellulose, or close-loop recycling systems to separate and reuse water, gas, and chemicals in the manufacturing process.
On the raw material side, cellulose is also found in straws, bagasse, bamboo, the cell walls of green algae, and the membranes of most fungi. New technologies allow these materials to be used to replace wood and cotton in cellulose fiber production. An example is the China-based Qingdao Yuanhai New Material Technology launched the world’s first commercialized alginate fiber plant in 2019, with an annual capacity of 5,000 metric tons. Their alginate fibers are used for nonwoven, garments, medical applications, etc.
Cellulose-like fibers are also developed to reduce the consumption of wood and cotton. Chitosan fibers and protein fibers are among these examples. Chitosan is a chemically cellulose-like biopolymer, which is processed from chitin, the world’s second largest natural polymer only after cellulose in terms of annual production volume. Chitosan fibers are bio-based, biodegradable, non-toxic, and biocompatible. However, they also have limitations such as poor mechanical and thermal strength. Currently, several companies have built chitosan fiber production facilities at scales of hundreds of metric tons annually.
Bio-based Synthetic Fibers
Bio-based synthetic fibers are produced by chemically or biologically synthesizing renewable organic materials. Polylactic acid (PLA) was the first bio-based synthetic fiber, first synthesized more than 150 years ago. But it was not commercialized until the late 1980s and early 1990s when DuPont and Cargill explored applications for lactic acid, lactide and PLA. Cargill first produced PLA in a 6,000 metric tons per year plant in 1994 and developed a continuous process for high purity lactide production based on reactive distillation.
Currently the global bio-based synthetic fiber market is dominated by polytrimethylene terephthalate (PTT) fibers, polylactic acid (PLA) fibers, polyethylene terephthalate (PET) fibers, and polyamide (PA) fibers. But polybutylene succinate (PBS) fibers, polyhydroxyalkanoates (PHAs) fibers, and several others also show promise. PLA, PBS, PHAs are biodegradable, while PTT, PA, and PET non-biodegradable.
Based on the projections from European Bioplastics e.V. and other sources, bio-based synthetic fibers could have a strong growth of around 25% per year from 2022 through 2027. But they had a market size of only 200,000 to 300,000 metric tons by 2022, much smaller than regenerated fibers (above 7.5 million metric tons) and bio-based natural textile fibers (approximately 27 million metric tons). Based on the above information and analysis, rough estimates can be made that the global fiber industry could produce approximately 3 million metric tons more regenerated fibers, 2 million metric tons more bio-based natural fibers, and 0.5 million tons more bio-based synthetic fibers in 2027 than in 2022.
Polylactic Acid (PLA) Fiber
PLA is derived from agricultural products with monomers that can be produced by microbial fermentation. It is well suited for melt spinning into fibers. Compared with the solvent process used for synthetic cellulosic fibers, the melt spinning process is less expensive and produces better properties. PLA fibers are nontoxic and fully biodegradable, reverting to their basic constituents: carbon dioxide (CO2) and water.
European Bioplastics e.V. forecasts that the global capacity of PLA polymers will have a compound annual growth rate (CAGR) of 39% from 460,000 metric tons in 2022 to 2.38 million metric tons in 2027 as a result of a series of large-scale new facilities built in Asia, North America, and Europe. Approximately 10% of the capacity, or 50,000 metric tons per year, was for fiber application in 2022. Historically, the fiber segment grew a little slower than the whole PLA market, and if this trend continues, PLA fibers could grow at a CAGR of 30%-35% from 2022 to 2027 and reach a capacity between 186,000 and 224,000 metric tons per year.
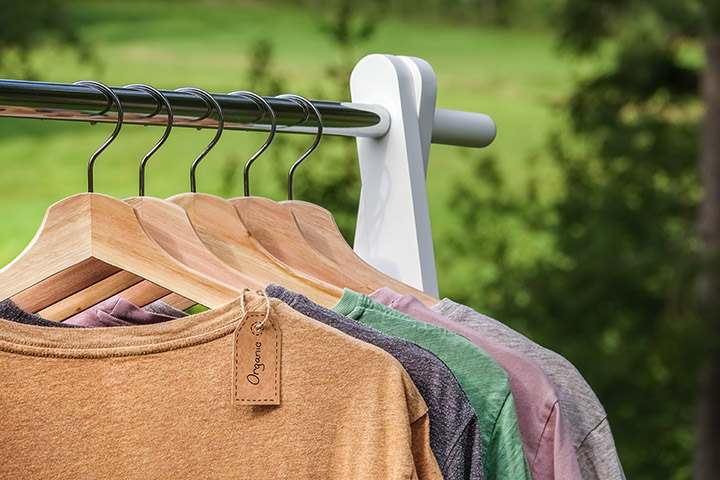
Unmodified PLA has several limitations such as brittleness, especially below 45ºF, and low heat distortion temperatures (HDTs). Strong market perspective has driven innovations for PLA modification, mainly through three routes: chemical modification, physical modification, and compatibilizer modification.
Chemical modification methods include block copolymerization, graft copolymerization, cross-linking, and chain extension for improving the tensile strength, tensile modulus, thermal stability, and biocompatibility of PLA fibers. Physical modification uses thermoplastics (e.g., poly ether-ether ketone), biodegradable resins (e.g., polycaprolactone), elastomers, rubbers, nano-particles, block polymers, graft polymers, or other fibers to improve the tensile strength, tensile modulus, thermal stability, crystallinity, biodegradability, biocompatibility, and elongation at break. Compatibilizers (e.g., PBS and ethylene glycol methacrylate) are used to improve the tenacity of PLA fibers.
In addition to the above three modification methods, technological innovations for PLA fibers also focus on the following segments: the technologies of fermentation, separation, and purification for PLA raw material, lactic acid; the green dyeing technologies for PLA fibers and yarns; the design for industry-scale melt-spinning facilities; and additional properties to PLA fibers such as flame retardance and antibacterial performance.
Polyhydroxyalkanoates (PHAs) and Polybutylene Succinate (PBS) Fibers
PHAs are biodegradable in soil and aquatic environments. The most prominent commercially available PHAs are polyhydroxybutyrate (PHB) and polyhydroxybutyrate hydroxyvalerate (PHBV). Currently, the U.S.-based Danimer Scientific and companies in China, Germany, Italy, and Brazil are key suppliers to PHAs.
Danimer Scientific estimated the PHA market could have a potential of 230 million metric tons per year. However, today the PHA industry is still in its infancy, with a global capacity of around 50,000 metric tons per year. Moreover, fiber accounts for only a fraction of PHAs applications. In 2022, the world’s total production of PHAs fibers was less than 1,000 metric tons.
Fiber manufacturers are currently pushing the use of bio-based PTT in high-end garments, home textiles, and industrial textiles.
PHBV fibers are biocompatible and biodegradable, which make them ideal materials for making medical textiles. Currently, the China-based Tianan is the largest PHBV supplier. However, PHBV fibers have several limitations such as brittleness, poor thermal stability, and being difficult to process. Currently, researchers are using block copolymerization, graft copolymerization, physical modification, and other methods to solve these problems.
PHB, the simplest PHA with properties similar to polypropylene (PP), is produced by bacteria that process glucose or starch. The Germany-based Biomer extruded their resin into multifilament fibers for woven surgical patches.
On the other hand, PBS is a bio-based polyester with similar properties to PET. It has excellent mechanical and biodegradable properties and good thermal stability and is easy to process, which allow it to be used in a wide range of end applications ranging from food packages to hygiene products. Currently, the commercialization for PBS fiber is still in the early stage. However, it is a promising product worthy of note for the following decade.
Non-biodegradable Bio-based Synthetic Fibers
The non-degradable bio-based synthetic fiber market is dominated by bio-based PTT fibers, followed by bio-based PA fibers and PET fibers. While the expansion for bio-based PTT fiber and PET fiber stagnates, the main driver for the capacity growth is bio-based PA fiber.
Today, the bio-based PA market is dominated by petrochemical giants such as BASF, DuPont, and Arkema. In 2007, BASF introduced Ultramid Balance nylon 6/10 which is based on approximately 60% sebacic acid, a material derived from castor oil. In 2009, DuPont introduced the Zytel product family which includes nylon 10/10 and nylon 6/10 along with their copolymers and their alloys with other polymers. DuPont’s nylon 10/10 contains 100% sebacic acid content while its nylon 6/10 contains 63%. China’s Cathey joined the competition in 2021 with an annual capacity of 100,000 metric tons of PA56, which is based on 45% renewable sources.
Currently the global production capacity for bio-based PA resins was more than 200 million metric tons if measured at the level of their bio-based content. Around 40 thousand metric tons are used for fiber production. Based on the current expansion plans, the global capacity of bio-based PA fibers is very likely to exceed 100,000 metric tons per year by 2027.
On the other hand, PTT is linear aromatic polyester made from 1, 3-propanediol (PDO). The bio-based edition of PTT was first commercialized by DuPont when the company launched Sorona, a PTT fiber based on bio-based 1, 3-PDO made through fermenting biomass sugars and glucoses.
Currently, bio-based PTT fiber is the largest bio-based synthetic fiber, with a capacity of over 200,000 metric tons a year. On the other hand, bio-based PET is mostly used for plastic bottles, and only a little more than 10,000 metric tons go to fiber production. Key manufacturers of bio-based PTT include the U.S.-based DuPont, and the China-based Shenghong and Glory.
Fiber manufacturers are currently pushing the use of bio-based PTT in high-end garments, home textiles, and industrial textiles. PTT provides good stretch recovery, softness and dyeability to garments, as well as excellent resilience, wearability, dyeability, static resistance, and chemical resistance to home textiles and industrial textiles.
Today, there is an overcapacity in the bio-based PTT and bio-based PET resin industries. Therefore, there is no large-scale bio-based PTT and bio-based PET resin projects planned for the next few years, though their fiber applications could still have significant growth in the same period.