Figure 1. Micrographs showing the effects of oxygen plasma treatments on polypropylene fibers after 30 and 60 seconds. A. SEM images of treated fibers. B. ESEM images of water droplets on treated fibers.
By Martin McCoustra and Robert Mather, Heriot-Watt University and Power Textiles Ltd.
Gas plasma treatment technology will be a game changer for processing textiles, and in particular technical textiles. The treatments adapt their surface properties without affecting any of their bulk properties. The plasma technique provides a clean, dry approach that consumes far less energy than do traditional wet treatments, the level of effluent is greatly reduced and, crucially, equipment on an industrial scale is commercially available(1). Depending on the type of plasma treatment, hydrophilicity or hydrophobicity can be altered, flame retardant properties can be enhanced, good adhesion between fibers and matrix can be promoted in composites and laminates, and many other desirable application properties can be promoted. A particularly important advantage provided by plasma treatments is the greatly increased scope for grafting polymers to a textile surface, so that the surface can be tailored to suit the intended application for the textile. This capability provides scope for new properties not achievable by conventional finishing treatments. So what are plasma treatments, and how much do we really know about them?
Nature of Gas Plasmas
Gas plasmas are complex mixtures of excited molecules, positive and negative ions, free radicals and electrons. A plasma is therefore a highly complex mixture of species, all of which can interact with one another and with the textile surface. Everyday examples of plasmas include fluorescent lamps and lightning flashes. During plasma treatments energy is supplied continuously, or in pulses, by means of an electrical discharge, either at low pressure in a vacuum chamber or in an atmospheric pressure plasma device.
The nature of the surface modification achieved depends on a variety of operating control factors, such as the composition of the gas, textile construction, the temperature and duration of the treatment, and the power and frequency of the electrical supply. Several effects of plasma treatments can be broadly identified. One effect is etching or cleaning of a fiber surface, and induces changes in surface texture. Alternatively, the surface may be chemically modified through the introduction of chemical functional groups. Wettability, adhesion and even biocompatibility can be improved, or by contrast, fiber surfaces can be rendered more inert. Still another, and often very important effect is the deposition of very thin films of polymer onto fiber surfaces – so-called plasma polymerization. These films consist of highly cross-linked polymeric structures, which can confer improved surface properties.
It is evident then that plasma technology offers huge advantages as a textile processing strategy. There is therefore a clear need to understand how this technology actually functions, in order that practical processing conditions can be better formulated, with the need for fewer trials to achieve a desired result. Right now in textile technology, plasma treatments are largely “recipe” based. The technical textile industry would be much better served by improved understanding of the complex mechanisms involved. What are the species comprising a particular plasma, how do their concentrations change during treatment of a textile, and how do the textile surface chemistry and topography change? A judicious combination of microscopic and spectroscopic techniques, statistical methodologies and computer modeling can go a long way to resolving these questions.
Microscopic and Spectroscopic Techniques
Changes to topography can be identified using scanning electron microscopy (SEM) and atomic force microscopy (AFM) but it should be noted that microscopy involves the local analysis of only a very small area of just a single fiber. SEM is very well established, though evacuation to very low pressure is required. An important advance is the development of environmental scanning electron microscopy (ESEM), which can be used at ambient pressure. For example, changes in fiber wetting behavior resulting from plasma treatment can be directly observed, as shown in Figure 1. AFM is a probe microscopy that can also image fiber surfaces at ambient pressures, even in water! Moreover, the technique can provide quantitative measurements of surface mechanical properties by use of so-called force-distance curves, yet this capability has so far been little used with technical textiles. Plasma treatments are very likely to change surface mechanical properties, and AFM could provide a means of monitoring these changes. The changes could alter abrasion resistance, for example, and affect sensory aspects of fabric handle.
Fiber surface chemical changes can be followed using mass spectrometry (MS), especially secondary ion mass spectrometry (SIMS), X-ray photoelectron spectroscopy (XPS) and infra-red (IR) spectroscopy1,2. Both XPS and MS require evacuation of the sample. SIMS is useful for identifying the chemical constituents of a textile surface, though less reliable for determining their relative proportions, as the proportion of ions discharged from the surface varies with different ions. XPS is a useful adjunct to SIMS, for it provides a quantitative measure of surface elemental composition. IR spectroscopy is performed under atmospheric conditions. Individual surface chemical functional groups can be detected, and specific bonding characteristics can be determined. The potential of nanoscale IR coupled with mechanical measurements at that scale opens new windows correlating the chemical and mechanical. Figure 2(A) shows the topography of a polystyrene/low-density polyethylene (PS/LDPE) blend. The different moduli of the two constituents are highlighted in Figure 2(B), and IR also distinguishes the two materials, Figure 2(C).
Despite the valuable insight that can be gained from direct examination of plasma treated textile surfaces, further useful information is gained by following changes in the plasma device as treatment proceeds. The characterization of species within a plasma device goes back 20 years, but so far the techniques involved seem to have made little impact on textile technology. One particularly important technique for the in situ analysis of plasma species is quadrupole MS. Characterization of plasma species in situ is therefore now well established and could be more readily extended to textiles.
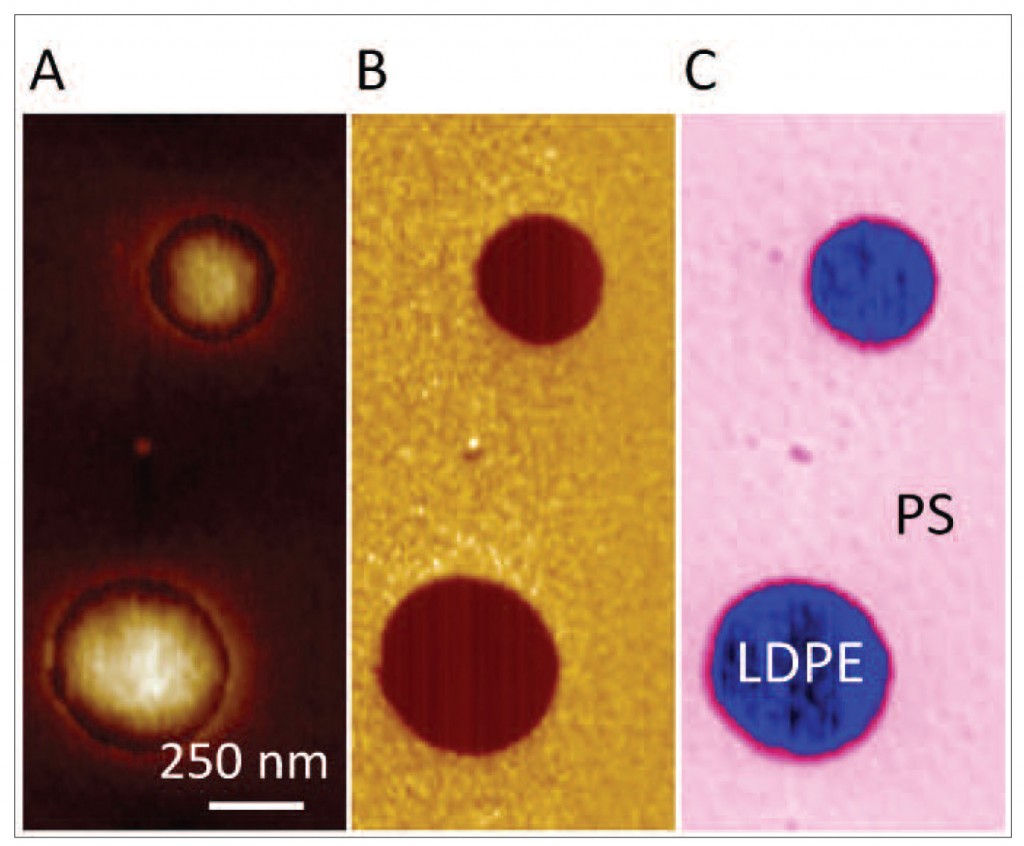
1880 cm-1 (C). Courtesy of Bruker Corporation
Statistical Methodologies
As stated above, there are a number of control factors determining the effects of plasma treatments on textile substrates. It is therefore advisable to conduct experiments on a sound statistical basis using factorial experimental design, so that key factors for a treatment, and any interactions between them, are reliably evaluated, using established statistical tests. Not only can optimum treatment conditions be better formulated, but important insights into the mechanisms will be gained.
Statistical methodologies are also now being applied to analysis of SIMS data obtained during the course of plasma treatments. For example, it can be the case that nearly all the information in the SIMS analysis is contained in just a few of the statistical components. As a result, particularly significant factors can be identified. These results can then usefully enhance those obtained in the standard identification of the key processing control factors.

Computer Modelling
Computer modeling has considerable potential to enhance textile plasma processing. At its simplest, a detailed understanding of the mechanisms and rates of plasma processing derived from detailed experimental measurements and computational simulation of textile materials has the potential to open new predictive process control pathways. The key agenda for the next generation of computational materials science is clearly bridging the gap between the quantum and atomistic worlds of materials science with the finite elements world of engineering. Therefore, while computer modeling of plasma treatments may seem highly theoretical to the practical textile technologist, it may well have its place as an adjunct to actual experimental approaches, provided the boundary conditions which limit all modeling approaches are recognized.
Conclusions
Plasma technologies will have huge impacts on commercial textile processing. Thus, Power Textiles Limited is using plasmas to enhance the chemical vapor deposition of silicon layers to develop photovoltaic fabrics3 (Figure 3). There is a real need to support the growth of plasma technologies with a much deeper understanding of them at a mechanistic level. Here, we advocate a variety of approaches that can be used in combination for the benefit of textile technology.
REFERENCES
1. R. Shishoo (editor), 2007. “Plasma technologies for textiles,” Cambridge, UK: Woodhead Publishing Ltd.
2. R.N.S. Sodhi, 2004. “Time-of-flight secondary ion mass spectrometry (TOF-SIMS): versatility in chemical and imaging surface analysis,” Analyst, 129, 483-487.
3. Robert Mather and John Wilson, April 2015. “Solar textile fabrics,” International Fiber Journal, 14-18.